Created: 6.05.2025 | 10:32 Updated: 6.05.2025 | 10:32
when we talk about atoms, we usually think of invisible spheres that make up everything around us. But how do they really behave when no one observes them? For decades, quantum physics has had to describe its movements from mathematical models and statistical averages, without being able to see what they do in real time. This limitation has changed with a recent experiment carried out by a team from the Massachusetts Technology Institute (MIT), which has achieved something unpublished: photograph individual atoms while interacting freely in space.
This work, published in the magazine Physical Review Lettersnot only represents a technical milestone, but also offers A direct window to the quantum worldallowing to see phenomena that until now existed on paper. Through an advanced atomic microscopy technique, MIT scientists have been able to observe how Bosonic atoms tend to group and how fermions, on the contrary, are paired at a short distance. It is the first time that these quantum correlations are visualized with atomic resolution in a continuous environment.
Quantum microscopy: stop the time to see the invisible
One of the main challenges when studying atoms is released is that They are never still. Its random movement and their behavior governed by quantum mechanics make it impossible to know where they are and how they move at the same time. To solve this problem, the MIT team developed a technique that allows them freeze the position of atoms during a second fractionjust before capturing a high resolution image.
The procedure is to let a cloud of atoms interact freely into an optical trap formed by lasers. At the exact moment of the registration, a light grid is activated – known as pinning lattice– which immobilizes atoms in their positions. Next, a second laser briefly illuminates them and captures their fluorescence through a cooling system by Raman side bands. This combination allows you to obtain individual atom images without significantly disturbing its original configuration.
As the authors indicate in the article, “We demonstrate the detection with atomic resolution of itinerant quantum bosons ²³na and ⁶li fermions, which allows direct measurement in situ of correlations between particles”.
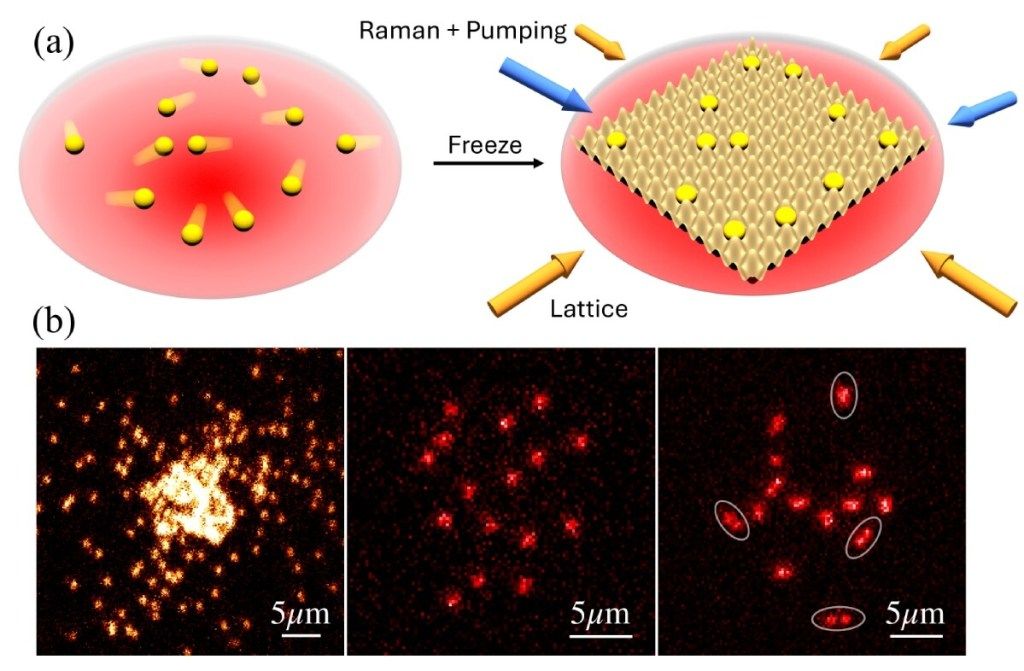
Bosones: Quantum grouping visible for the first time
In its first application, the technique was used to study A cloud of sodium atomswhich are bosons. Bosons, unlike other types of particles, They tend to occupy the same quantum state. At extremely low temperatures, this behavior results in a phenomenon known as Bose-Einstein condensatewhere all atoms act as a single quantum entity.
Thanks to the new microscopy, it could be clearly observed how bosons are grouped with a greater probability of being close to each other. This effect, known as “bunching”had been predicted since the time of Louis de Broglie, but had never been seen directly in real time and in the real space. According to the authors, “We reveal the Bose-Einstein condensation with a single atom resolution”an achievement that makes a key difference with respect to the previous methods of visualization by absorption.
In addition, researchers They measured the increase in second order correlations G (2)that quantify the probability of finding particle pairs together, and that in the case of bosons show a clear tendency to grouping.
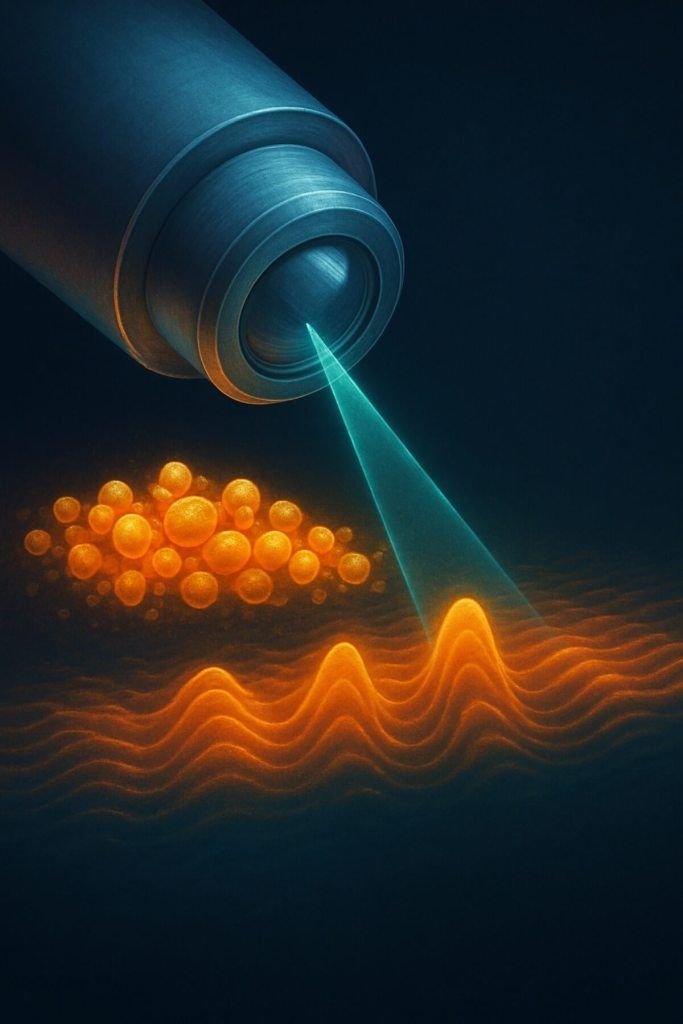
Fermions: The footprint of the exclusion principle
Fermions behavior, such as lithium-6 atoms used in the experiment, is very different. These particles They obey Pauli’s exclusion principlewhich means that they cannot share the same quantum state if they have the same spin. In practice, this makes They tend to get away from each othera phenomenon known as “Fermi hole” or fermi hollow.
In the MIT experiment, the team used a balanced mixture of two spin states of Lithium-6. In this context, they observed not only the effect of repulsion between particles of equal spin, but also The formation of pairs between opposite spines fermions. This interaction is essential for phenomena such as superconductivityand until now it had been indirectly inferred by spectroscopic techniques. Now, for the first time, it could be seen directly. “We observe pairs of non-local fermions at the BEC-BCS cross”, Indicates the article.
The technique also allowed to measure key parameters of these peers: the size of the torque, the pairing gap (pairing gap) and short -range contact (short-range contact), All of them essential to understand the physics of strongly correlated systems.
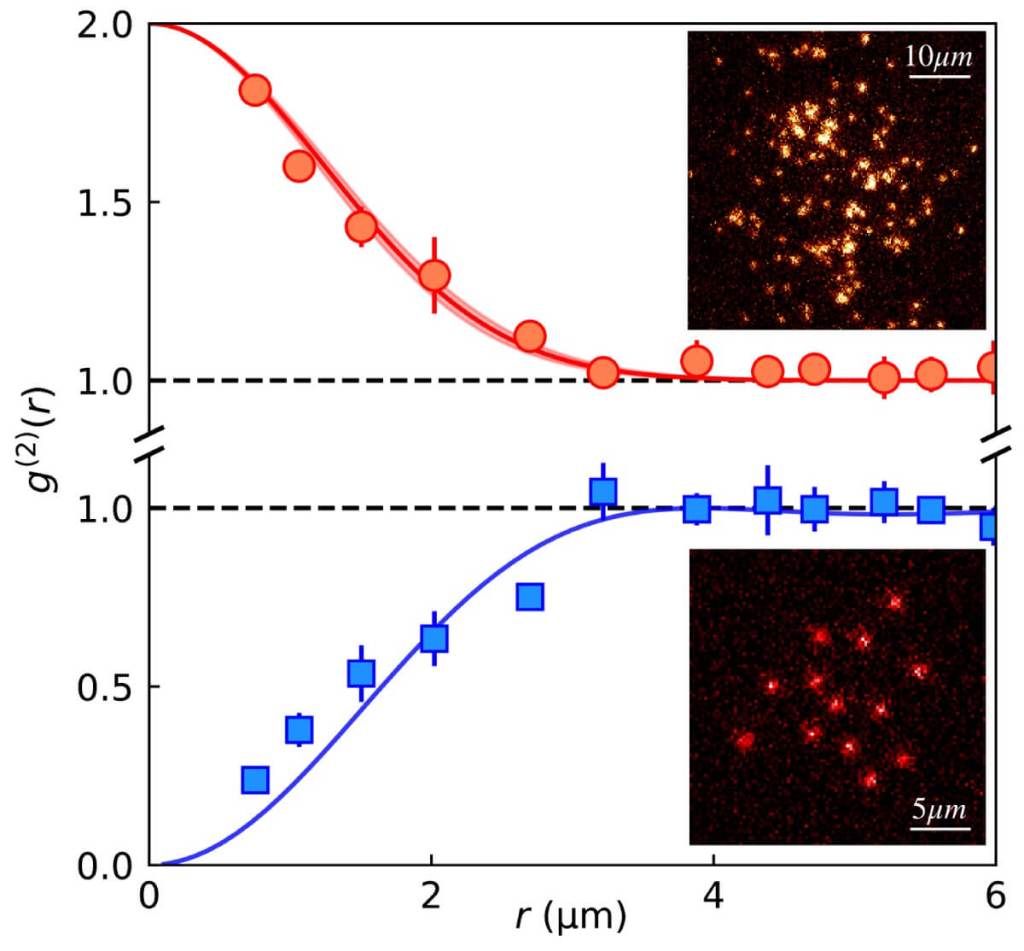
The quantum continuum without a network: a key difference
Most previous experiments on quantum gases were performed in reticular structures, similar to a network of regular optical traps. This design facilitated the capture of atoms, but also limited their natural behavior. The great novelty of the current study is that the atoms interact in the “continuous”that is to say, Without a previous confinement patternwhich better reflects its real quantum status.
The authors explain: “Unlike previous works with gases trapped in a network, here we perform microscopy of quantum gases in the continuum”. This extra freedom allows us to observe more complex systems, such as those that appear in the condensed matter or in the topological phases, with unprecedented fidelity.
In addition to allowing the direct study of the correlations between particles, this technique also opens the door to measure thermodynamic properties such as density, compressibility and pressureall with atomic resolution.
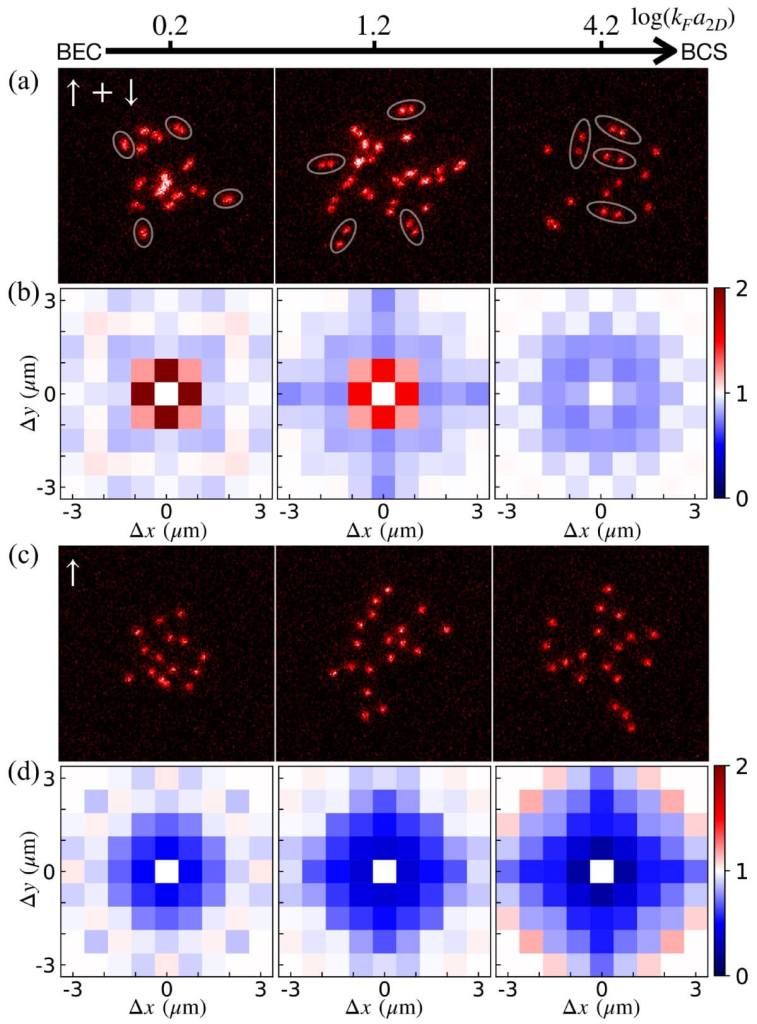
Towards a detailed map of the quantum world
One of the most powerful aspects of the experiment is that The technique can be applied to other systems. Researchers highlight their potential to study more exotic phases, such as fermions gases with spin imbalance, mixtures of bosons and fermions, dipole gases, or even systems with topological and superfluous characteristics.
This type of microscopy could become an essential tool for the call Quantum simulationa line of research that seeks to reproduce in laboratory the behavior of complex materials through cold atoms. Instead of building a theory from scratch, the quantum system is built and observed directly.
In addition, the results of the MIT coincide with those of other teams, such as those led by Wolfgang Ketterle in MIT and Tarik Yefsah himself in the École Normale Supérieure of Paris, which confirms the robustness and reproducibility of the data obtained. In this sense, the scientific community now has a new tool to study matter in its most fundamental state.
References
- Ruixiao Yao, Sungjae Chi, Mingxuan Wang, Richard J. Fletcher, Martin Zwierlein. Measuring pair correlations in Bose and Fermi gases via atom-resolved microscopy. Physical Review Letters (2025). https://doi.org/10.1103/PhysRevLett.XXX.